Everything researchers should know about lab reagents
The Research Reagent Paradox: A Process Review
Research Reagent Paradox
Introduction
The topic of material and supply has been a mystery to many in the world of life science research. Mostly to the researchers who aren’t traditionally required to care about such distractions. For years, several support departments called procurement, vendor management, etc. have been the standard for delegating these responsibilities away from the scientists. However, things have changed in the past decade with the help of ecommerce, the commercialization of basically all laboratory research material has been commoditized directly to the end-user. In other words, researchers are now doing their own shopping of laboratory supplies-especially their critical reagents.
Critical reagents can be a range of biomolecules produced for research-use, and express special characteristics. Often in their genetic or sequence specificity, these reagents are referred to as “analyte-specific”. Examples include recombinant proteins or monoclonal antibodies (very popular) and nucleic acid sequences of DNA/RNA or their short strand oligo-form. Critical reagents are much more sophisticated products compared to other laboratory consumables.
Many researchers begin experiencing the similar patterns in handling reagents. Our background as end-users, suppliers, and producers enables our team to utilize our insight to make sense of these patterns through what we refer to as, The Research Reagent Paradox.
Research Reagent Paradox
The research reagent paradox is a diagram analyzing components connecting manufacturing, supply-chain, and scientific research. This serves as a novel approach for analyzing, combining, and interpreting relatively complex intricacies into a big picture design. The research reagent paradox aims to reinforce every scientist and research professional with proper understanding of the reagents they depend on.
The diagram applies a simplified view of a molecules journey to a reagent and features the unique role data plays in transforming the process into a cycle. By focusing on stages of manufacturing, selling, and applying a research reagent; our schema can illustrate how data leverages a strong feedback effect that deserves focus in center stage.
In this paper, we present brief summaries of significant factors in each stage and conclude with a case for optimizing the dynamic.
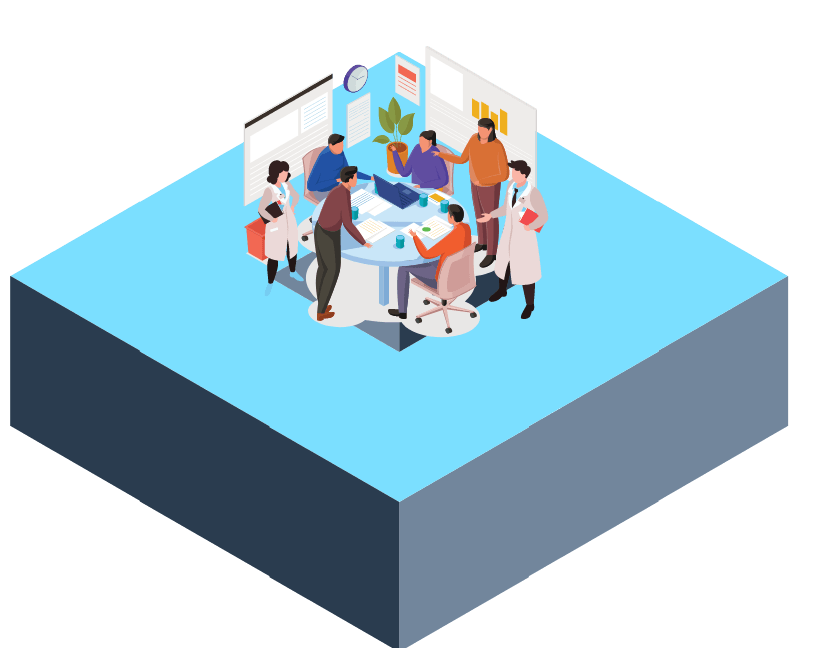
Current Data Dilemma
Data. What’s most precious inside a research laboratory loses significant consideration as moves away from the bench. In chemical manufacturing, data technology is generally low. With reagents, its low on both sides of the industry. Decades of scientific data being generated in different computing formats and no updates to legacy records has resulted in very rich scientific databases drowning in errors. Many of which serve as standard registries for their topic of focus. Whether it’s nucleic acid sequences, protein profiles or chemical libraries, the inconsistency and lack of uniformity in these databases has been a growing issue in use as reliable reference data. Additionally, the recent boom in bioinformatics further complicates the issue. The recent influx of computer scientists in life science research is historic. However, these new additions come pioneering their own data revolution in emerging and exciting fields such as the “complex-omics”. The rapid generation of their new sophisticated data is considerably more compelling and threatens to eclipse the issues of legacy databases. With minimal attention as is, decreased awareness further risks procrastinating a solution.
We want to be cautious with discussing data as it is easy to crossover to the topic of software and similar challenges in bioresearch. This is not about software or software engineering. Following sections will touch on some software components in relative terms. However, our focus is specific to data and its application.
Next, we will discuss how data translates into the beginning stage of reagent manufacturing and continues.
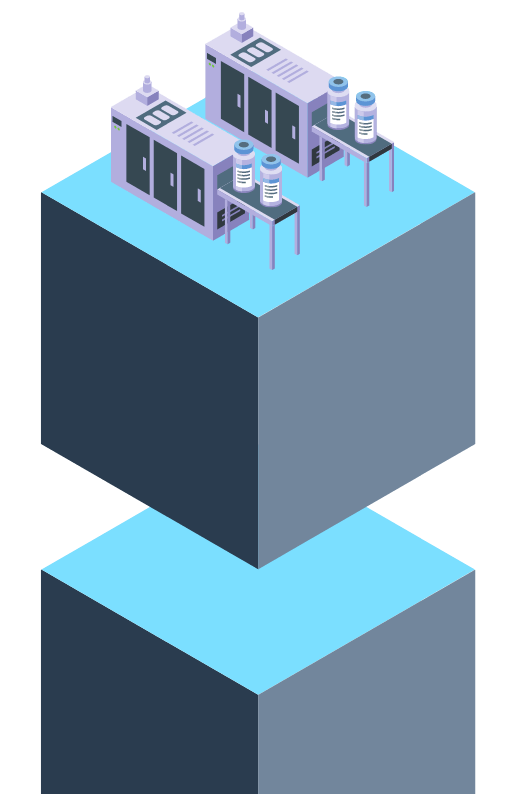
Stage 1. Production
Transforming an agent (molecule, chemical, formula, etc.) into a reagent begins with the production step. To clarify, the term “reagent” refers to the former (‘agent’) in the shape of a product. In other words, a reagent is the synthetic version of a certain biological molecule. Such molecules include nucleic acids, enzymes, antibodies, and other proteins- expressing specific genetic characteristics. Although this concept is generally understood, not many are familiar with the underlying development process and challenges-as is the case with many commodities. However, research reagents are different. The argument can be made that the commoditization of such complex material has been a leading factor to many of the manufacturing issues- faced today. To comprehend this, we must first understand the basics and state of specialty reagent manufacturing.
Production systems rely on the legacy databases as much as researchers, but in differing application. Almost all data required to design a manufacturing blueprint for reagents can be found in their respective registries. The planning time and labor saved is significant enough to rationalize against preliminary in-house validation. The approach of feeding these unvalidated blueprints into production systems has become common practice. Errors are then exposed throughout the process workflow. To counter errors, facilities use costly in-house troubleshooting by way of empirical methods and chemical trialing. The repeat success of troubleshoots optimize the initial blueprint and bring rise to a new practice of exclusive in-house formulations and in-house protocols. Unlike open-source data, the true value of these protocols is their inclusion of process variables and expert knowledge which often comes from analytical experience. This information cannot be calculated through modeling or computational methods. Its competitive gating further widens the gap between software and manufacturing.
Surrounding factors don’t leave much choice for production teams. Biomanufacturing systems are not versatile by nature. The equipment is insanely expensive/complex, the software is archaic, and there’s minimal automation between steps. This makes them incompatible to periodic innovation and impractical to renovate from scratch. Not to mention, manufacturing facilities are often running on an as-needed workforce. Meaning limited personnel – regularly overseeing a multitude of simultaneous production runs. What engineers have been able to achieve thus far using such raw data in suboptimal conditions is remarkable. It’s hard to ignore the potential with more flexibility and data integrity involved. For now, this system works in the sense that its capable of delivering a reagent in product form for research application. As a product, reagents become commercially available to research.
The next section provides an overview of how the marketplace operates. Continue reading as this process picks up in the hands of its sellers and buyers.
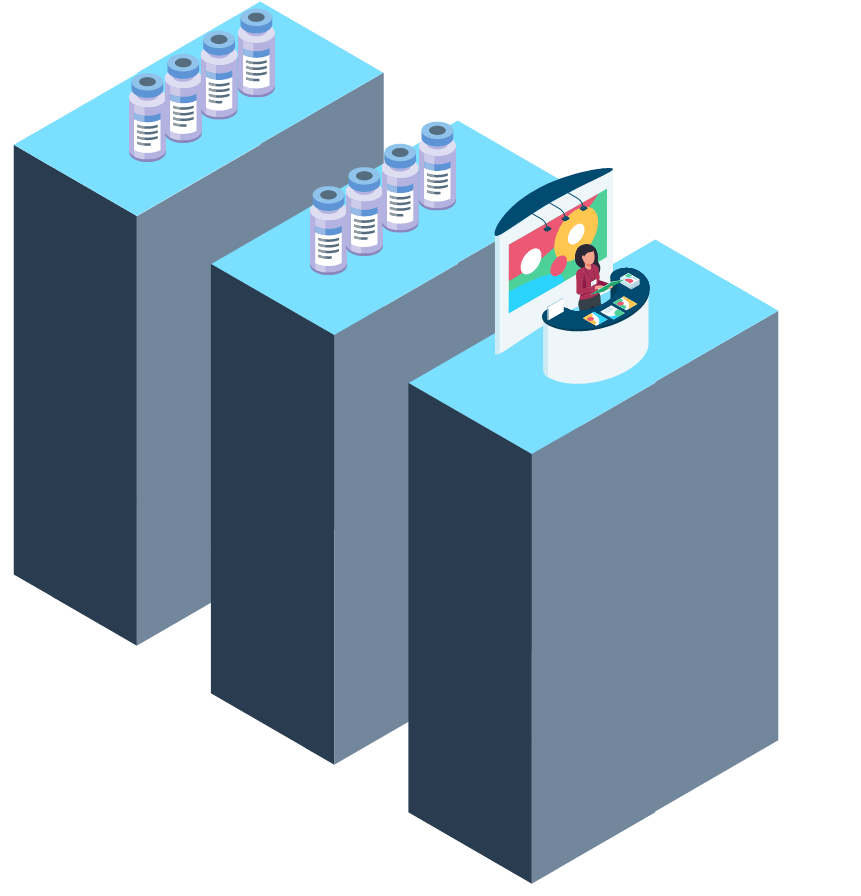
Stage 2. Supply Chain
Supply chain is its own phenomena in life science. We refer to it as the wild west of life sciences. An arena filled with manufacturers, distributors, 3rd party resellers, foreign entity sales hubs and everything in-between! None of which are outlaws, technically. The term “wild west” refers to the untraditional and often confusing exchanges between these companies in order to meet demand. The landscape plays by its own economics. With a shared objective of getting a product or service into research, science finds a way to reject market principles and complicate the supply process like no other industry. As proud members of this landscape, we have seen and experienced this on the frontline. Having to support both an in-house R&D model along with a supplier origin, we can keep the insight relevant to reagents.
It is important to understand that when a reagent arrives to supply chain it is considered a final product. In other words, most datasheet specs are final and not designed to be modified. Doing so would be costly for most sellers and disrupt their cost model-which is often based on inventory logistics. However, scientific research is very specific. Seldom does a researcher approach procurement by asking what’s available and basing experiments off those options. The need is specific. They need specific analytes that meet specific parameters for specific features to try and achieve specific results. With the inability to modify final products, the popular approach has been to build extensive catalogs filled with thousands of analyte reagents in varying forms with diverse features. An overwhelming attempt at presenting a higher probability of compatibility to such specific demand. Thus, it’s impossible to manufacture this many reagents under one roof. Proven by what we’ve discussed so far; co-op, cross-selling deals between every and any company that can help meet a researchers criterion becomes natural practice. Although, counterproductive over time as many companies find themselves sharing identical catalogs from similar suppliers -who in random cases are reselling from resellers. One principle that does apply here is the increase in cost and lead time as a product exchange hands. This makes sense to why prices of reagents vary so drastically from different companies. With no standard pricing in place, the list price for a small-scale reagent (10uL-1mg) can range from hundreds to thousands of dollars depending on the supplier agreement.
Consequently, the growing trends of current supplier models are affecting the industry in more ways than price and lead time. With many catalogs selling reagents via ecommerce “add-to-cart” and little to no engagement of the end-user with the source manufacturer, product feedback quality remains poor. Feedback of how a reagent performs against different applications is critical for optimizing its blueprint. Data such as results under certain experiment conditions or in combination with other agents has been historic to developing new products. However, the online buying convenience and debased buyer communication model limits the level of value supply chain can offer research. While an efficient approach to meet short-term needs for small scale reagents, it proves unsustainable when a reagent enters scale-up demand for long term use. We’ve yet to see the latter be achieved without diligent communication.
Ultimately, the goal of supply chain is to get the product into the buyers’ hands which in this case is the “end-user”. It is generally agreed that the success of this is defined by your reagent performing well in an end-user’s research. This baseline is key for transitioning into the final stage of the reagent paradox. All roads lead to the end-user’s research.
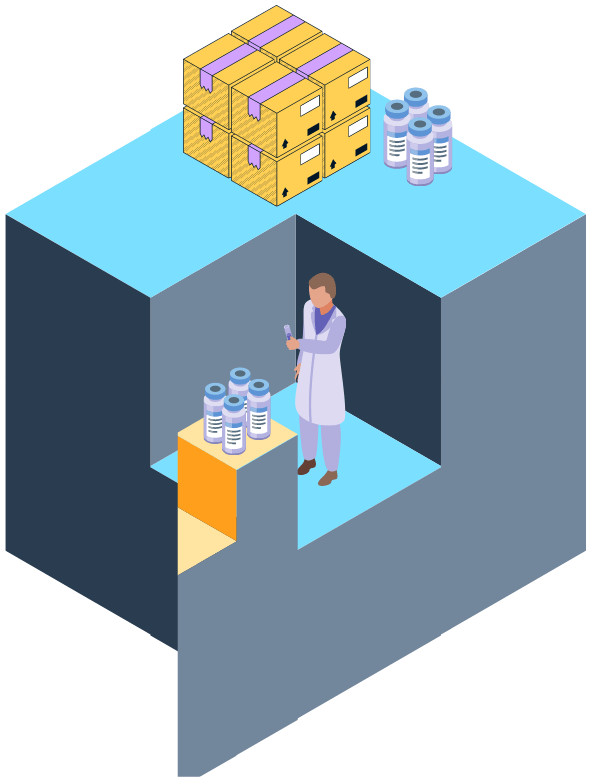
Stage 3. End-User
The scientific end-user is the ultimate destination for any research reagent. Reagents are a critical for running experiments and driving results. Consequently, data produced from these results plays a novel role in our paradox. One might assume that a reagents journey ends once finally consumed by the end-user. We would argue that the journey, in fact does not end; but instead, proceeds into a cycle. Consider the prerequisite sharing practices of scientific research. Bulk of research data is distributed through journal publication or similar. As well as less formal methods such as posters, legacy databases, etc.
In any case, data is presented via strict guidelines which includes the experiment details. An essential section titled “materials & methods” is where a reagent lives on. For many years the materials section allowed scientists to effectively discover and source new reagents. It acted as a feedback system for performance and incentivized better production.
Although this is no mystery, it’s value has become underrated as search engines and other 3rd parties quickly become the initial procurement tool. Materials and methods also serve as a quality reference for manufacturers to help tailor products to various applications and optimizing reagent formulation. Thus, contributing to the initial manufacturing stage keeping the cycle constant.
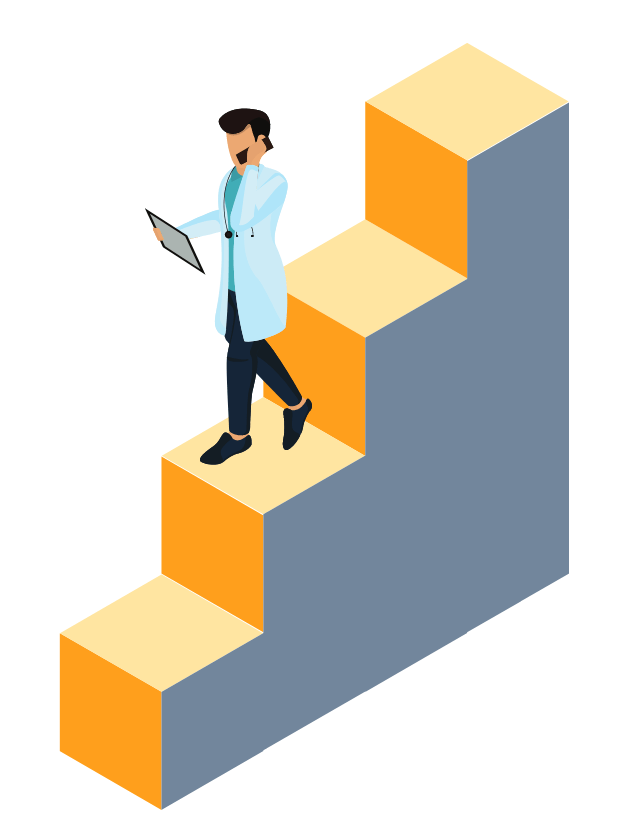
Concluding Analysis
The factors discussed for each stage are specific to the paradoxical dynamic of the process. With the analysis of scientific database deficiencies, production innovation limits, disconnects in supply-chain and the ultimate role of end-user’s research data – we provide insight on hindering factors to support a unique perspective for the value of reagents in scientific research. This allows process illustration of the significant stages throughout reagent development to its application via “The Research Reagent Paradox”. Emphasis on oversight of data and the misalignment of values at each stage suggest key areas of focus going forward.
In addition to greater focus, our diagram also serves as an immediate resource to researchers or similar buyers of reagents. The information can be essential in building a competitive sourcing strategy for laboratories. We recommend utilizing it to optimize due diligence when sourcing a reagent. In our experiences, this is the best way to minimize compromise and risk of experimental error,
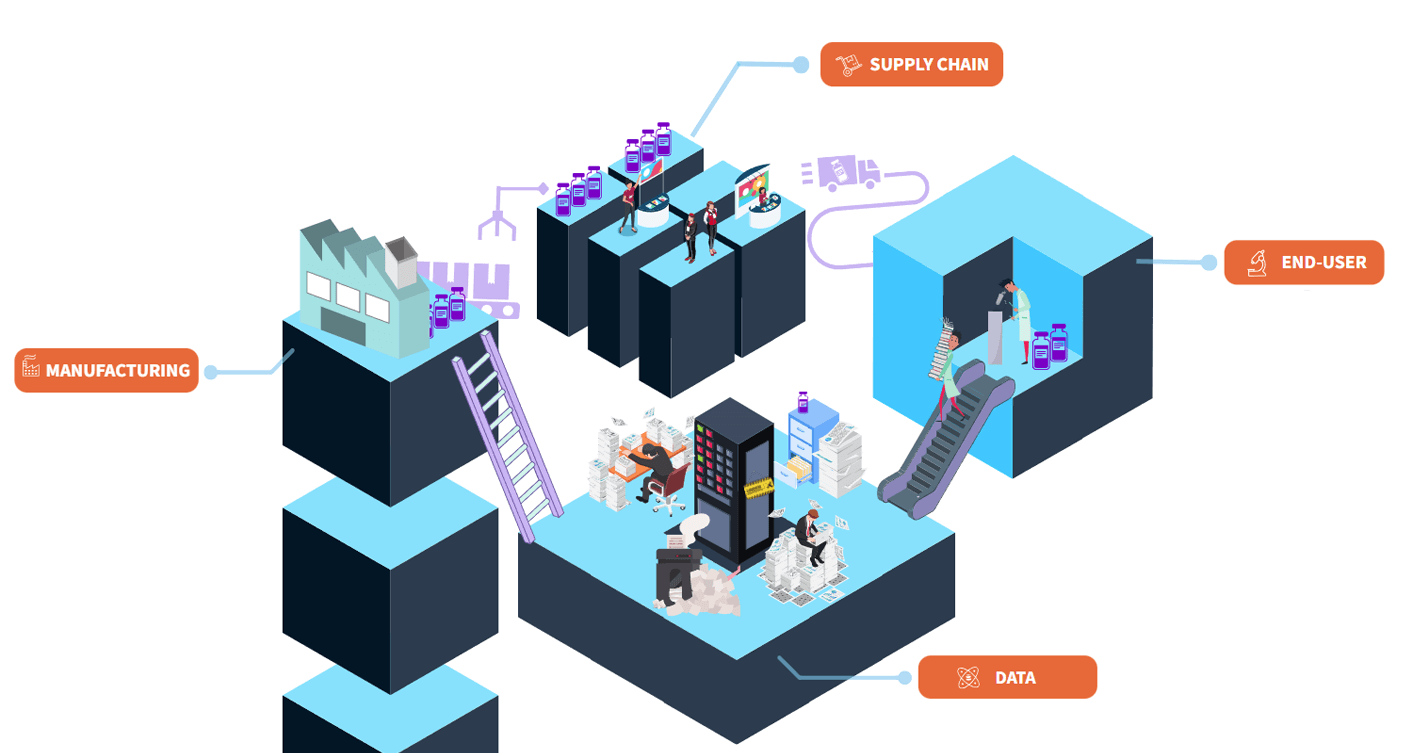
References
____________________________________________________________________________________________
C.F. Mandenius
Recent developments in the monitoring, modeling and control of biological production systems
Bioprocess Biosyst Eng, 26 (2004), pp. 347-351
J.E. Bailey
Mathematical modeling and analysis in biochemical engineering: Past accomplishments and future opportunities
Biotechnol Prog, 14 (1998), pp. 8-20
A.S. Rathore, R. Bhambure, V. Ghare
Process analytical technology (PAT) for biopharmaceutical products
Anal Bioanal Chem, 398 (2010), pp. 137-154
M. Stojcev, T. Tokic, I. Milentijevic
The limits of semiconductor technology and oncoming challenges in computer micro architectures and architectures
Facta Univ - Ser Electron Energ, 17 (2004), pp. 285-312
H. Narayanan, et al.
Bioprocessing in the Digital Age: The Role of Process Models
Biotechnol J, 15 (2020), pp. 1-10
W. Sommeregger, et al.
Quality by control: Towards model predictive control of mammalian cell culture bioprocesses
Biotechnol J, 12 (2017), pp. 1-7
S. Shioya, T. Takamatsu, K. Dairaku
Measurement of State Variables and Controlling Biochemical Reaction Processes
IFAC Proc, 16 (1983), pp. 13-25
M. Kishimoto, T. Sawano, T. Yoshida, H. Taguchi
Application of a statistical procedure for the control of yeast production
Biotechnol Bioeng, 26 (1984), pp. 871-876
J.H. Lee
Model predictive control: Review of the three decades of development
Int J Control Autom Syst, 9 (2011), pp. 415-424
A predictive high-throughput scale-down model of monoclonal antibody production in CHO cells
Biotechnol Bioeng, 104 (2009), pp. 1107-1120
S. Nargund, K. Guenther, K. Mauch
The Move toward Biopharma 4.0
Genet Eng Biotechnol News, 39 (2019), pp. 53-55
Accelerating biologics manufacturing by modeling or: Is Approval under the QbD and PAT approaches demanded by authorities acceptable without a digital-twin?
Processes, 7 (2019), pp. 1-28
Pinu, F. R., Beale, D. J., Paten, A. M., Kouremenos, K., Swarup, S., Schirra, H. J., & Wishart, D. (2019). Systems biology and multi-omics integration: Viewpoints from the Metabolomics Research Community. Metabolites, 9(4), 76. https://doi.org/10.3390/metabo9040076
Manzoni, C., Kia, D. A., Vandrovcova, J., Hardy, J., Wood, N. W., Lewis, P. A., & Ferrari, R. (2016). Genome, transcriptome and proteome: The rise of OMICS data and their integration in Biomedical Sciences. Briefings in Bioinformatics, 19(2), 286–302. https://doi.org/10.1093/bib/bbw114
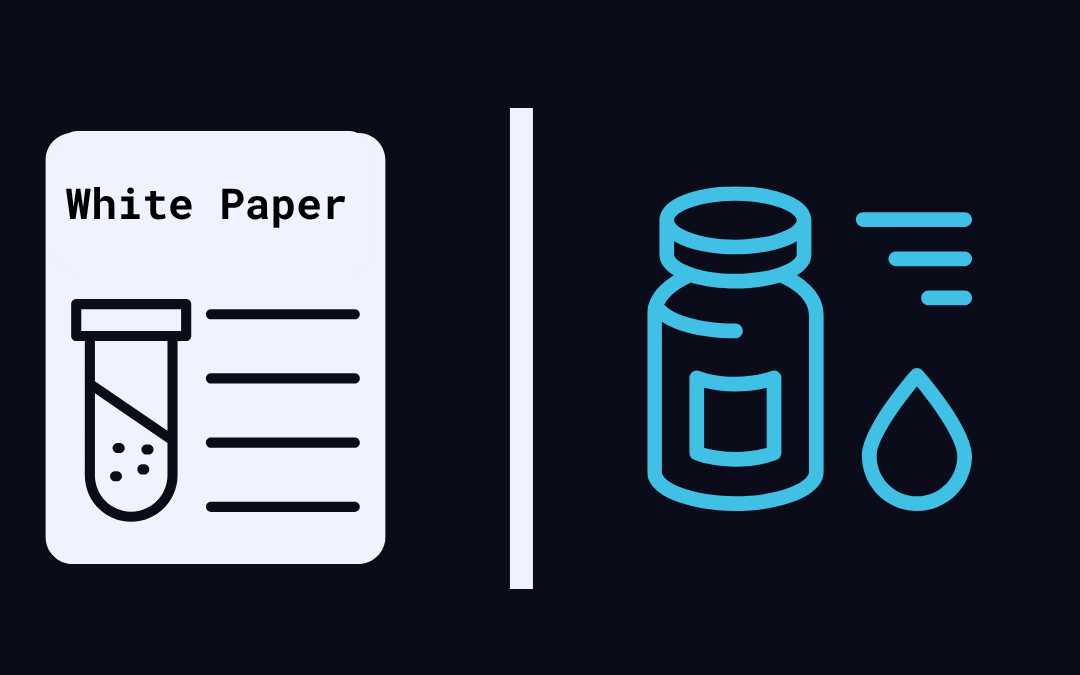
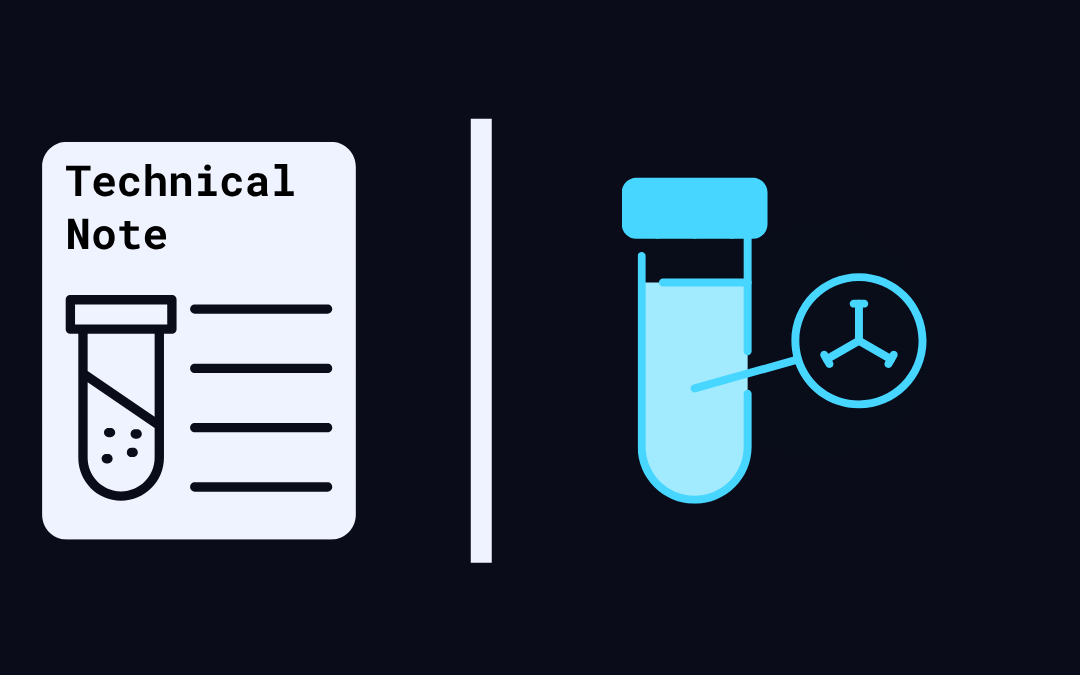